AIR-INDEPENDENT
PROPULSION
AIP
Technology Creates a New Undersea Threat
by Edward
C. Whitman
|

Walter Type XVIIB up on
the stocks. Pictured
above is the German Walter Type XVIIB U-1406, partially
dismantled shortly after the end of World War II. U-1406
was turned over to the U.S. Navy as a war prize and soon
disposed of, but the Royal Navy later operated her sister ship, U-1407,
as HMS Meteorite to gain experience in hydrogen-peroxide propulsion technologies.
|
As
interest mounts in "Air-Independent Propulsion" (AIP)
for enhancing the performance of small, defensive submarines, a
serious new underwater threat is developing in littoral waters.
Increasingly, smaller nations unwilling or unable to accept the
high cost of nuclear power to achieve greater underwater
endurance and longer range are turning to lower-priced and less
ambitious alternatives that still offer significant operational
advantages over conventional diesel-electric submarines. The
best of the latter boats, such as the German-designed Type 209
or the Russian KILO, can remain submerged on battery at slow
speed for periods on the order of three to five days. But now,
several AIP schemes in development or already in operation can
increase slow-speed endurance to as much as three weeks or a
month. While still dwarfed by the potential of nuclear power,
AIP offers diesel submarines a remarkable increase in capability.
|
AIP - The Early
History
Despite their initial successes, submarine pioneers were still eager to
find some means to free their boats from the necessity of surfacing
frequently for access to the atmospheric oxygen demanded by the gasoline
or diesel engines that charged the batteries. A number of approaches
were tried, but eventually, open-cycle diesel engines, lead-acid
batteries, and electric motors for submerged propulsion became the
standard submarine engineering plant that served well through two world
wars.
In the early 1930s, however, a brilliant German engineer, Dr.
Helmuth Walter (ca. 1900-1980) of Kiel's Germaniawerft, proposed
a radical new submarine propulsion plant based on the use of high-purity
hydrogen peroxide (H2O2) as an oxidant. In Walter's system, hydrogen
peroxide from an onboard supply was decomposed using a permanganate
catalyst to yield high temperature steam and free oxygen. Into the
reaction chamber was injected diesel fuel, which combusted with the
oxygen to yield a mixture of steam and hot gas that drove a high-speed
turbine. The exhaust and condensed steam were then expelled overboard.
Walter's primary design goal was high underwater speed, rather than long
endurance, and indeed, his first submarine prototype, the experimental V80,
reached 28.1 knots submerged in its 1940 trials - at a time when
conventional submarines were limited to 10 knots or less. Thus, V80,
only 76 tons and 22 meters long, also served as an early test bed for
studying the dynamics and control of high-speed underwater vehicles.
Later in the war, the Kriegsmarine attempted to scale Walter's
prototype up to a useful operational size, but although seven Type XVIIB
H2O2 coastal boats were completed before Germany's final defeat, none
saw combat. These Type XVIIs displaced 300 tons and were powered by two
2,500 horsepower turbines, in addition to a conventional diesel-electric
plant. More ambitious plans to build larger Walter-designed ocean-going
submarines, such as the 800-ton Type XXVI and the 1,600-ton Type XVIII
were thwarted by the unsuccessful course of the war and the realization
that the industrial capacity needed to supply sufficient quantities of
hydrogen peroxide could never be achieved. However, the Type XVIII was
modified into the highly successful Type XXI "electro-boat,"
in which larger batteries provided a submerged speed of 17 knots, which
could be maintained for 90 minutes. That innovation, and the adoption of
the snorkel, yielded a potent combination that strongly influenced the
postwar design of conventionally-powered submarines on both sides of the
Iron Curtain.
AIP Fallout from
World War II
After the conflict, several nations sought to exploit Dr.
Walter's revolutionary propulsion concepts. As war prizes, the United
States and Britain received the scuttled Type XVIIBs, U-1406 and U-1407,
respectively, and the latter was resurrected for experimental purposes
as HMS Meteorite. Additionally, Walter himself and several of his
key staff were brought to England and there collaborated with Vickers,
Ltd. for several years in the design of more advanced hydrogen peroxide
systems. The result was two 1950s-era high-speed boats, HMS Explorer
and HMS Excalibur, whose design was heavily influenced by that of
Walter's wartime Type XXVI. While both boats achieved stated design
goals for high underwater speed, their highly-concentrated hydrogen
peroxide fuel created such a safety hazard that the two boat became
known as "HMS Exploder" and "HMS Excruciator." Both
were decommissioned in the 1960s.
The Soviet Union built a single, semi-
successful exemplar of a Walter-cycle boat, known in the West as
"the Whale," but their most serious AIP efforts were focused
on a closed-cycle diesel plant based on the German Kreislauf
system and their own researches prior to the war. Eventually, this led
to the 650-ton Soviet QUEBEC class (1956) that used stored liquid oxygen
to sustain closed-cycle operation for diesel engines on three shafts.
Although 30 were built between 1953 and 1957, their safety record was so
dismal that they were known by their crews as "the cigarette
lighters" and withdrawn from service by the early 1970s.
Meanwhile,
the United States had salvaged a 2,500-horsepower Walter turbine from U-1406,
as well as a 7,500-horsepower version planned for the Type XXVI, and set
them up at the Naval Engineering Experiment Station at Annapolis,
Maryland. Subsequently, the Navy funded research on several alternative
submarine AIP approaches, including variants of the Walter-cycle and Kreislauf
systems. Eventually, unacceptable growth in the required size and weight
of the corresponding engineering plants - plus the growing prospect in
the late 1940s of submarine nuclear propulsion - soon brought these
efforts - and those of the British and Russians - to a close. USS Nautilus
(SSN-571) got "underway on nuclear power" in January
1955.
X-1 – The U.S.
Navy's First Midget Submarine
However, in September 1955, the U.S. Navy's first midget submarine, the
one-of-a-kind X-1 (SSX-1), was launched on Long Island with a
closed-cycle hydrogen peroxide/diesel plant! Inspired by the success of
the British "X-craft" of World War II, X-1 was intended
for shallow-water commando operations. Displacing 36 tons submerged on a
length of some 50 feet, X-1 was powered by a heavily modified
commercial diesel engine with a small battery-powered electric motor as
a backup. On the surface, the ambient atmosphere charged the engine, but
underwater, the oxygen required for combustion was derived from the
catalytic decomposition of hydrogen peroxide in a reaction chamber. Both
engine exhaust and water condensate were compressed and discharged
overboard. Four hundred gallons of peroxide could be
stored in a flexible polyvinyl-chloride bag forward, and the craft could
accommodate four crewmembers.
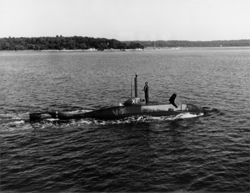
|
(left) X-1
Midget Sub. The U.S. Navy's 1955 mini-submarine X-1 used a
rudimentary AIP system in which oxygen for underwater operation of
its conventional diesel engine was derived from the decomposition
of highly concentrated hydrogen peroxide. After a peroxide
explosion in 1957, however, its AIP capability was eliminated. |
(right) HMS
Explorer at sea. One of two experimental Walter-turbine
submarines built by the British in the 1950s, HMS Explorer
achieved her designed underwater speed, but suffered enough
engineering mishaps to earn the nickname, "HMS
Exploder." |
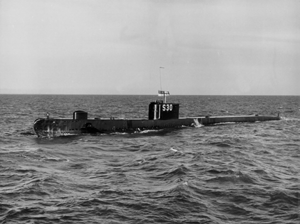 |
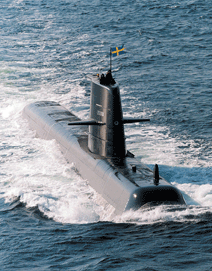
|
(left) Gotland
Class. The Swedish Navy's three Gotland-class
submarines each use two Stirling cycle engines as an adjunct to
their main diesel-electric engineering plants to provide
underwater endurance up to several weeks. This was the first AIP
system to enter regular submarine service. |
After several engine failures and
subsequent design modifications, X-1 finally achieved acceptable
performance in February 1957 and undertook a series of operational
trials based at the Portsmouth Naval Shipyard. Unfortunately, in May
1957, an explosion in the hydrogen peroxide storage system blew off the
whole bow section, and although no one was injured, X-1's
closed-cycle capability was never replaced. Instead, the boat was
rebuilt with a small, conventional diesel-electric/battery plant, and
after being laid up for three years, it was reactivated in late 1960 and
subsequently used until 1973 for a variety of research studies in the
Chesapeake Bay. Later, X-1 was put on static display at the U.S.
Naval Academy in Annapolis, and more recently at the Nautilus Museum in
Groton, Connecticut. Significantly, her former Officer-in-Charge later
wrote, "The most important lesson learned from this experimental
program was… that high concentration unstabilized hydrogen peroxide
has no place on a fighting ship.
Current Efforts
in AIP
Although major naval powers like the United States, the United Kingdom,
and the Soviet Union turned quickly to submarine nuclear propulsion as
soon as it became technically feasible, smaller navies have remained
committed to conventional diesel-electric submarines, largely for
coastal defense. Many of these have incorporated innovations originally
pioneered in the German Type XXI, but more recently, growing demand for
longer underwater endurance has generated increasing interest in
promising AIP technologies, both old and new. Currently, system
developers are actively pursuing the following generic approaches for
achieving "closed cycle" operation:
- Closed-cycle diesel engines, generally
with stored liquid oxygen (LOX)
- Closed-cycle steam turbines
- Stirling-cycle heat engines with
external combustion
- Hydrogen-oxygen fuel cells
Closed-cycle
Diesel Engines
Typically, a closed-cycle diesel (CCD) install- ation incorporates a
standard diesel engine that can be operated in its conventional mode on
the surface or while snorkeling. Underwater, however, it runs on an
artificial atmosphere synthesized from stored oxygen, an inert gas
(generally argon), and recycled exhaust products. The engine exhaust -
largely carbon dioxide, nitrogen, and water vapor - is cooled, scrubbed,
and separated into its constituents, with the argon recycled back to the
intake manifold. The remaining exhaust gas is mixed with seawater and
discharged overboard. Generally, the required oxygen is stored in liquid
form - LOX - in cryogenic tanks.
CCD systems have been developed by a number of firms in Germany,
Britain, the Netherlands, and a few other countries. However, except for
a 300-horsepower demonstration system refitted onto the German Navy's
ex-U 1 in 1993, no modern CCD systems have entered naval service.
England's Marconi Marine recently acquired CCD pioneer Carlton Deep Sea
Systems and is marketing a CCD retrofit package for existing
conventional submarines, such as South Korea's nine Type 209s. Although
one key advantage of CCD systems is their relatively easy backfit into
existing submarine engineering plants, there have been no takers.
Despite the additional supply complication of needing regular
replenishment of cryogenic oxygen and inert gas, there are logistics
advantages in retaining standard diesel engines and using normal diesel
fuel.
Closed-cycle
Steam Turbines
The only steam-turbine AIP under active investigation is the French
MESMA system (Module d'Energie Sous-Marin Autonome). This is
essentially a conventional Rankine-cycle turbo-alternator powered by
steam generated from the combustion of ethanol (grain alcohol) and
stored oxygen at a pressure of 60 atmospheres. This pressure-firing
allows exhaust carbon dioxide to be expelled overboard at any depth
without an exhaust compressor.
Basically, the MESMA approach is a derivative of French
nuclear-propulsion experience using non-nuclear steam generation.
Although MESMA can provide higher output power than the other
alternatives, its inherent efficiency is the lowest of the four AIP
candidates, and its rate of oxygen consumption is correspondingly
higher. The first full-scale undersea application will be in Pakistan's
three new Agosta 90B submarines, which will each be fitted with a 200
kilowatt MESMA system for increasing submerged endurance by a factor of
three to five at a speed of 4 knots. The first installation is expected
to be completed in 2001.
Stirling-cycle
Engines
In the Stirling cycle, heat from an outside source is transferred to an
enclosed quantity of working fluid - generally an inert gas - and drives
it through a repeating sequence of thermodynamic changes. By expanding
the gas against a piston and then drawing it into a separate cooling
chamber for subsequent compression, the heat from external combustion
can be converted to mechanical work and then, in turn, to electricity.
Like MESMA, this approach has an advantage over internal combustion
systems, such as the CCD, in that the combustion processes can be kept
separate from those that actually convert heat to mechanical work. This
provides significant flexibility in dealing with exhaust products and
controlling acoustic radiation.
The Stirling-cycle engine forms the basis of the first AIP system to
enter naval service in recent times. The Swedish builders, Kockums Naval
Systems, tested a prototype plant at sea in 1989, and today, three
Swedish Gotland-class boats are each fitted with two adjunct, 75
kilowatt Stirling-cycle propulsion units that burn liquid oxygen and
diesel fuel to generate electricity for either propulsion or charging
batteries within a conventional diesel-electric plant. The resulting
underwater endurance of the 1,500-ton boats is reported to be up to 14
days at five knots, but significant burst speeds are possible when the
batteries are topped up.
Fuel Cells
In simplest terms, a fuel cell is an electrochemical conversion device
that combines hydrogen and oxygen to produce water, electricity, and
heat. Fuel cells are already seeing a number of promising applications
in the space and automotive industries, and many authorities believe
that fuel cells offer the best potential for developing more capable AIP
systems in the future. There are several alternative configurations, but
for submarine propulsion, so-called "Polymer Electrolyte
Membrane" (PEM) fuel cells have attracted the most attention
because of their low operating temperatures (80° Centigrade) and
relatively little waste heat. In a PEM device, pressurized hydrogen gas
(H2) enters the cell on the
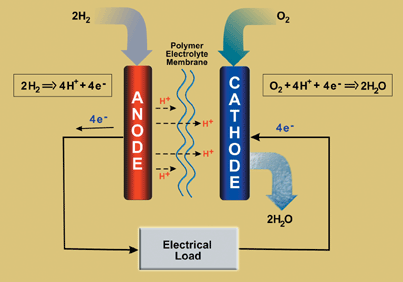 |
In a typical fuel cell, gaseous
hydrogen and oxygen are combined catalytically to produce water,
heat, and useful electricity. Already successful in the U.S.
space program, fuel cells are seeing increasing use as submarine
power sources. |
anode side, where a platinum catalyst
decomposes each pair of molecules into four H+ ions and four
free electrons. The electrons depart the anode into the external circuit
- the load - as an electric current. Meanwhile, on the cathode side,
each oxygen molecule (O2) is catalytically dissociated into
separate atoms, using the electrons flowing back from the external
circuit to complete their outer electron "shells." The polymer
membrane that separates anode and cathode is impervious to electrons,
but allows the positively-charged H+ ions to migrate through
the cell toward the negatively charged cathode, where they combine with
the oxygen atoms to form water. Thus, the overall reaction can be
represented as 2H2 + O2 => 2H2O,
and a major advantage of the fuel-cell approach is that the only
"exhaust" product is pure water. Since a single fuel cell
generates only about 0.7 volts DC (direct current), groups of cells are
"stacked" together in series to produce a larger and more
useful output. The stacks can also be arrayed in parallel to increase
the amount of current available.
The greatest challenge for fuel-cell AIP systems lies in storing the
reactants. Although oxygen can be handled with relative safety as LOX,
storing hydrogen onboard as a liquid or high-pressure gas is very
dangerous. One solution is to carry the hydrogen in metal hydride
accumulators, at low pressure and ambient sea temperature. (A metal
hydride is a solid compound of hydrogen and metallic alloy, in which
individual hydrogen atoms occupy interstitial positions in the host
metal's crystalline lattice. By manipulating temperature and pressure,
hydrogen gas can be absorbed or released at will.) Another, less
efficient, approach is to generate gaseous hydrogen from a stored liquid
hydrocarbon such as diesel fuel, kerosene, or methanol. This requires an
auxiliary device called a "reformer," in which a mixture of
hydrocarbon and water is vaporized and superheated under pressure to
yield a mixture of hydrogen and carbon dioxide.
Several manufacturers are currently offering fuel cell systems for
submarine AIP. Prominent among these is the German Siemens firm,
which is collaborating with Howaldtswerke Deutsche Werft (HDW)
and Italy's Fincantieri to supply fuel cell installations for the
forthcoming 1,840-ton German and Italian U 212-class submarines.
These will consist of nine PEM fuel-cell modules each nominally rated at
34 kilowatts, to yield a total of approximately 300 kilowatts (400
horsepower). With metal-hydride hydrogen storage, the system is
predicted to yield 14 days submerged endurance and the ability to run up
to eight knots on the fuel cells alone. Siemens is working on a
next-generation PEM module rated at 120 kilowatts, and two of these will
be incorporated into HDW's 1,860-ton U 214 boats, planned as
export successors to the U 212 series. Other nations, such as
Russia and Canada - the latter with significant under-ice requirements -
are also considering fuel-cell modules for either new construction or
for upgrading older boats.
Other key advantages here are both higher efficiency and lower specific
stored-oxygen consumption than the other alternatives.
An AIP
Perspective
Although it is a remarkable tribute to Hellmuth Walter's engineering
genius that he fielded a fully functional - if troublesome -
5,000-horsepower AIP system in 1945, the maximum power output of current
AIP installations is typically on the order of 400 horsepower (300
kilowatts). In comparison, the conventional diesel-electric plant of the
U 212 class described above is rated at over 3,000 horsepower,
and a typical nuclear submarine propulsion plant produces over 20,000.
Since the power required to propel a submerged body varies with the cube
of its velocity, it should be apparent that at least for the near
future, AIP will be valuable primarily as a low-speed, long-endurance
adjunct to the under- water performance of conventional submarines.
There is little short-term prospect for AIP to become a primary,
full-performance alternative to either diesel or nuclear power. Even the
phrase "closed cycle" is something of a misnomer, because
except for fuel cells, all AIP alternatives require ejecting exhaust
gases overboard, which limits both depth capability and stealth.
However, this is not to minimize the dangerous potential for AIP
submarines to complicate seriously both coastal defense and assured
access to littoral regions. If their distinctive characteristics are
exploited by skillful operators, AIP submarines can be used to telling
effect for both short- and medium-range missions. AIP dramatically
expands the tactical "trade-space" for diesel-electric
submarines. If conditions permit, they can transit rapidly on the
surface with-out unduly expending the wherewithal for superior
underwater performance. Submerged, they can opt for a long, slow, silent
patrol that keeps their batteries fully charged and thus capable of
powering speed bursts of significant duration. And by carefully
husbanding their resources, they can revert again to slow-speed
operation and repeat the cycle several times over weeks of submergence.
Moreover, AIP technology is evolving rapidly, and some experts predict,
for example, that the power output of a typical fuel cell module could
well double or triple in the next several years, allowing an even more
advantageous trade-off between underwater speed and endurance.
Their tactical flexibility, their small size, their inherent stealth -
and the novel operational paradigms AIP submarines introduce to undersea
warfare - will make these new boats a dangerous threat to submariners
accustomed to nuclear- or conventionally diesel-powered adversaries. The
Submarine Force needs to understand this threat - where it's been, where
it's going, what it means, and how to counter it.
Dr. Whitman is the Senior Editor of
UNDERSEA WARFARE Magazine.
TABLE
OF CONTENTS
|